Additive direct-write manufacturing has evolved into an integral element in research and development due to its unique advantages and in particular due to its 3D printing possibilities. While matured down to the smallest micrometer range, the expansion to the real nanoscale is still a challenging task. Among the small pool of potential techniques [[1]], Focused Electron Beam Induced Deposition (FEBID) has matured from a trial-and-error laboratory method into a predictable 3D Nano-Printing (3DNP) technology with unique advantages [[2]].
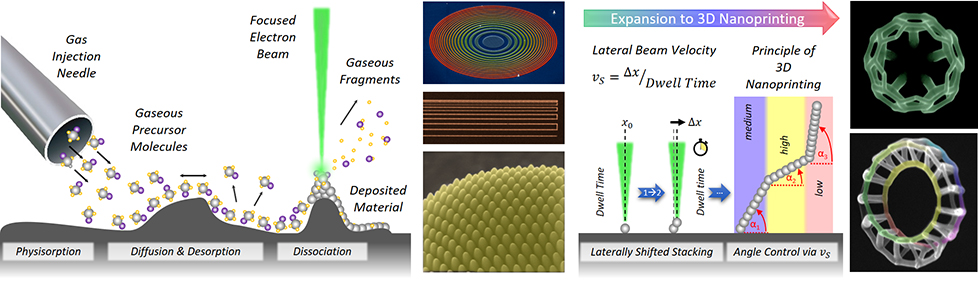
FEBID is typically performed in a Scanning Electron Microscope (SEM), which is equipped with a gas injection system (GIS) and an appropriate patterning engine for controlled beam movement. As conceptually shown in upper figure (left), functional precursor molecules are injected into the vacuum chamber, where they physisorb onto, diffuse along and eventually desorb from the surface. The interaction with the focused electron beam leads to a local dissociation into volatile and non-volatile fragments. While the former get pumped away, the latter are immobilized and form the intended deposit according to the precise beam movement. By that, this technology truly represents a highly flexible additive direct-write fabrication technology with resolution capabilities down the sub-20 nm regime, while demands on the substrate material and surface morphologies are very low. Even more importantly, FEBID enables the fabrication of complex, freestanding 3D nano-objects. The concept is shown in upper figure (right) and relies on systematic, laterally displaced stacking of nano-volumes, which allows single-step fabrication of even complex 3D nano-designs[2], as illustrated by several examples in the upper figure. To induce the required functionality, we use different approaches to tune morphologies [[3]], functional properties [[4]] or even transfer the materials in pure metals [[5]], which, for instance, is the key element towards 3D plasmonics [[6]].
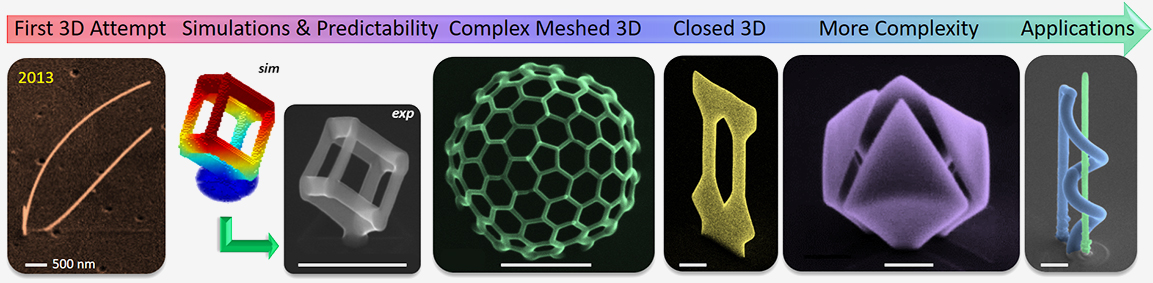
At FELMI-ZFE, FEBID has a long lasting history where we started with more planar structures, which 2013 turned into 3D nanoprinting (see figure below). On the one hand, we focus on the gradual expansion of design possibilities [[7]] by a close look on fundamental processes [[8]] together with technological aspects. That, in turn, leads to new combined approaches, which enable unique application concepts in 3D space for research [6,9]] but also for industry[[10]] as e.g. executed in the frame of the Christian Doppler Laboratory for Direct-Write Fabrication of 3D Nano-Probes (CDL-DEFINE).
[1] Additive Manufacturing of Metal Structures at the Micrometer Scale. Luca Hirt et al.; Adv. Mater. 2017, 29, 1604211.
[2] 3D Nanoprinting via Focused Electron Beams. Robert Winkler et al.; J. of Applied Physics 2019, 125 (21), 210901.
[3] Controlled Morphological Bending of 3D-FEBID Structures via Electron Beam Curing. Anna Weitzer et al.; Nanomaterials 2022; 12 (23), 42.
[4]Optimization of Postgrowth Electron-Beam Curing for Focused Electron-Beam-Induced Pt Deposits. Harald Plank et al.; J. Vac. Sci. Techn. B 2011, 29 (5) 051801.
[5] Rapid and Highly Compact Purification for Focused Electron Beam Induced Deposits: A Low Temperature Approach Using Electron Stimulated H2O Reactions. Barbara Geier et al.; J. Phys. Chem. C 2014, 118 (25), 14009.
[6] i) Direct-Write 3D Nanoprinting of Plasmonic Structures. Robert Winkler et al.; ACS Appl. Mat. Interf. 2017, 9 (9), 8233; ii) High-Fidelity 3D Nanoprinting of Plasmonic Gold Nanoantennas. David Kuhness et al.; ACS Appl. Mater. Interf. 2021, 13 (1), 1178.
[7] Expanding FEBID-Based 3D-Nanoprinting toward Closed High-Fidelity Nanoarchitectures. Anna Weitzer et al.; ACS Appl. Electr. Mat. 2022, 4 (2) 744.
[8] i) Shape Evolution and Growth Mechanisms of 3D-Printed Nanowires. Robert Winkler et al.; Additive Manufacturing 2021, 46, 102076; ii) 3D Nanoprinting Replication Enhancement Using a Simulation-Informed Analytical Model for Electron Beam Exposure Dose Compensation. Jason D. Fowlkes et al.; ACS Omega 2023, 8, 3, 3148.
[9] Tunable 3D Nanoresonators for Gas‐Sensing Applications. Georg Arnold et al.; Adv. Funct. Mat. 2018, 28 (19), 1707387.
[10] i) Focused Electron Beam-Based 3D Nanoprinting for Scanning Probe Microscopy: A Review. Harald Plank et al., Micromachines 2019, 11 (1), 48; ii) 3D Nanoprinting of All-Metal Nanoprobes for Electric AFM Modes. Lukas M. Seewald et al.; Nanomaterials 2022, 112 (24), 4477; iii) Three-Dimensional Nanothermistors for Thermal Probing. Jürgen Sattelkow et al.; ACS Appl. Mat. Interf. 2019, 11 (25), 22655.